Energy, conversion, detection
The PHOTO team covers a wide field of research applied to energy, photo-conversion and photo-detection. Our studies focus on the interactions between the elementary excitations, photovoltaic cells based on III-V semiconductors, hybrid systems for solar energy harvesting, thermophotovoltaic conversion, and hyperspectral imaging.
III-V semiconductor solar cells for space applications
Multi-junction solar cells (MJSC) based on III-V semiconductors are part of the third generation of photovoltaic cells. Our aim is to improve the quality of these materials and their conversion efficiency, taking into account their use in the space environment, in collaboration with our partners at ONERA and CNES. Our aim is to improve understanding and modeling of the behavior of materials (e.g. dilute nitrogen-based alloys such as InGaAsN, used in certain sub-cells, tel-03483437) and heterostructures used in novel multijunction solar cells used in non-standard environments such as space.
Hybrid systems for solar energy conversion
The conversion efficiency of photovoltaic solar systems is limited. In order to recover and convert solar energy optimally, we are working on several approaches to convert solar energy by hybridizing photovoltaic conversion with another type of conversion. Our main focus is on hybridization with thermoelectric generators.
- PV-TE hybridization
Depending on the photovoltaic material, up to 50% of the solar energy striking the cell is lost and dissipated as heat. An emerging strategy for harvesting this waste energy is to couple the solar cell with a thermoelectric generator (TEG) into a hybrid photovoltaic-thermoelectric (PV-TE) system. The first experimental realizations of hybrid PV-TE systems, though simple, show a promising gain in efficiency of up to +5%.
To go further, in the framework of the ANR HYDRES project, in collaboration with Institut Jean Lamour (Nancy), we propose a global and multidisciplinary approach to overcome the technological barriers hindering the development of highly-efficient hybrid PV-TE systems.
Specifically, in the Photonics team , we address two key challenges:
- functionalizing the interface between the solar cell and the TEG to boost the heat flow incoming in the thermoelectric module and its contribution to the output power of the PV-TE system (PhD thesis of Sébastien Hanauer, 2020-2023, hal-04020888);
- developing a technological process to fabricate a PV-TE device with a high thermal coupling (PhD thesis of Léopold Boudier, 2022-2025).
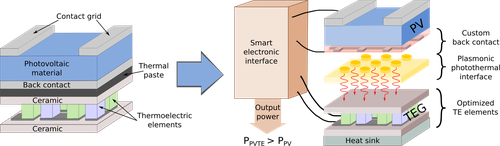
Towards an architecture of PV-TE hybrid system with high conversion efficiency (functionalization of the PV-TE interface, specific design of the TEG, implementation of a smart electronic interface for maximum power point tracking).
- Other PV-X hybridizations
Several approaches allowing a better use of solar energy by associating photovoltaic cells with another converter are possible. In particular, we are involved in collaborative research aimed at modeling, designing and implementing PV - CSP and PEC - PV hybrid converters.
In the case of hybridization with Concentrated Solar Power (CSP) technologies, where the solar radiation is concentrated on an absorber that allows a fluid to be heated at high temperature, used to produce electricity via turbines, we analyze several configurations of association with photovoltaic conversion. More specifically, our scientific contribution concerns the analysis of the behavior of PV cells when they are under stress (e.g. thermal stress when the cells cover the absorber of the solar power plant). This research is carried out in collaboration with PROMES (Odeillo) and RAPSODEE (Albi) laboratories in the frame of a thesis (Zacharie Menard, 2023-2026) funded by ANR (labex SOLTICE) and the Occitanie Region (PV-STAR).
In the case of photoelectrochemical (PEC) cells used to produce solar fuels (e.g. hydrogen), only the most energetic solar photons are used. Hybridization with PV cells operating in the near-infrared allows for better conversion of solar radiation, as the electricity produced contributes to the control of the PEC cell. We mainly participate in the analysis of the behavior of low-energy bandgap PV cells (~0.7 eV) under concentrated solar irradiance with truncated spectrum. The work is carried out in collaboration with Institut Pascal (Clermont-Ferrand) and LEMTA (Nancy), and more generally within the framework of an ANR project (MCMET, 2024-2028) aimed at implementing statistical methods (Monte Carlo) for a multi-physics and multi-scale modelling of this type of solar system.
Thermophotovoltaics
Thermophotovoltaic (TPV) conversion is based on the same principles as for solar photovoltaic conversion, but for a light source which is a hot body at high temperature (typically from 700 to 3000 °C). Our research activity mainly deals with proposing materials and structures for TPV cells and photonic components optimizing conversion performances.
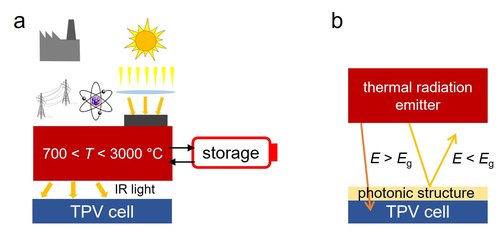
- Very-low bandgap (<0.36 eV) TPV cells
Theoretically, in order to maximize electrical power, it is required to use photovoltaic cells having a forbidden energy band (or bandgap) smaller than 0.6 eV for emitter temperatures below 3000 °C.
In the case of medium-grade thermal radiation sources (< 1000 °C), the requirement is stronger: cells with very low bandgap (from 0.17 to 0.36 eV) should be used. The main challenge is to make the cell operational at room temperature.
In the frame of an ANR funded project (LOW-GAP-TPV, 2021-2025), we participate to proposing materials and structures allowing very-low bandgap TPV conversion. In collaboration with IES (Montpellier), we have chosen to use an absorber made of InAs/InAsSb superlattice and a barrier structure.
- Architectures minimizing electrical and optical losses
Conversely, when the emitter temperature is very high (> 2000 °C), high bandgap cells (> 0.8 eV) can still be used. However, several challenges need to be considered.
As for concentrated solar photovoltaics, the cells are under high illumination conditions, and thus they generate high electrical currents. As a result, the Joule losses are becoming one of the main limiting factors. In collaboration with the University of Lleida (Spain), we explore the possibility to use silicon vertical multijunction cells originally developed for solar photovoltaics, hal-04186071. Thanks to a strong current reduction, resistive losses are negligible. The challenge is to modify the architecture of the cell for TPV conversion.
The second issue with using cells with bandgap larger than 0.8 eV is that a large fraction of the incident photons does not contribute to the photovoltaic effect. If these photons are absorbed, they will lower conversion efficiency and generate heat. It is therefore necessary to apply a spectral filtering for minimizing their absorption. Our research activity consists in designing and applying original solutions to optimize infrared light management in TPV cells. III-V materials with bandgap comprised between 0.8 and 1.1 eV are chosen for the absorber.
Interaction between elementary excitation and their hybridization
The vectors of energy conversion in its different forms (electromagnetic, vibrational, thermal) are the interactions between elementary excitations (excitons, plasmons, phonons, and polaritons). Our research focuses on understanding these interactions and manipulating them through the micron and nanometer size structure of matter. The hybridization of elementary excitations is giving rise to novel concepts like acousto-plasmonics, plasmo-electronics, plasmo-excitonics, and thermo-plasmonics. In addition to numerical simulations of optical responses and heat transport, we are applying experimental tools focused mostly on localized optical spectroscopy techniques (confocal micro-PL and micro-Raman, multi-spectral micro-reflectivity and transmission, ellipsometry). The areas of energy (capture and storage, photocatalysis, and hydrogen production) and the identification of chemicals or biological interest (LSPR sensing, SERS) are the focus of the targeted applications. The materials of choice are: plasmonic metals (aluminum, gold, silver), thermoelectric materials (BiTeSe), hybrid organic-inorganic perovskites (with high excitonic and dielectric confinement), two-dimensional semiconductors from the family of transition metal dichalcogenides (with high quantum confinement) and their heterostructures. To manage and improve these materials' optical, thermal, or vibrational properties, they are combined by chemical synthesis, epitaxy or deposition.
- Acousto-plasmonics
Most people view nano-objects as static objects. Actually, they are the center of vibrations in their atomic lattice, which are exclusively acoustic in the case of noble metals. The latter are linked to the objects' individual and collective electronic excitations and heavily rely on their dimensions and forms. Modelling these couplings is of relevance to acousto-plasmonics, which makes use of them to describe spectroscopic data (resonant Raman) and comprehend the transformation of electromagnetic energy into thermal energy.
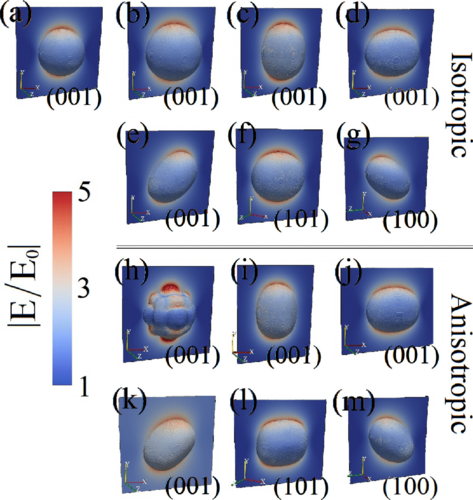
2D and 3D spatial distributions of the electric near field enhancement induced by the dipolar LSPR and modulated by the isotropic (top) and anisotropic (bottom) vibration modes ; the corresponding cross-section planes are indicated in each panel. (a) NP at rest, (b) isotropic breathing S100 (d0 ) mode, (c)–(g) fivefold degenerated isotropic quadrupoles S12m (d1 -d5 ), (h) anisotropic breathing A1g mode, (i), (j) anisotropic quadrupole Eg (E g(a) and E g(b) ) modes, and (k)–(m) anisotropic quadrupole T2g (T2g(a) , T2g(b) , and T2g(c) ) modes. Published in Phys.Rev.B; collaboration : Prof. Nicolas Large, UT San Antonio.
- Thermo-plasmonics
Plasmonic nanostructures' capacity to absorb, confine, focus, and guide light has opened up new avenues for research and applications in a variety of industries, including telecommunications, energy, and health. Our goals in the Photonics group are as follows: (i) Optimize emission, absorption, guiding, filtering, or optical focusing functions by integrating plasmonic functions into devices. (ii) create a multi-purpose method by taking advantage of metallic nanostructures' capacity to conduct current, produce thermal hot spots, and transmit light selectively. (iii) employ two-dimensional quantum wells and plasmonic metasurfaces to enhance the photo-generation of charge carriers for photovoltaic and photocatalytic uses.
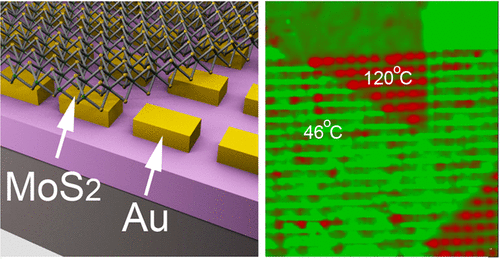
Thermo-plasmonics experiment in a hybrid nanostructure composed of gold nano-antennas coated with a monolayer of MoS2. The nanoantennas absorb light efficiently, converting it into electron-hole pairs that release their excess energy by emitting phonons. Published in ACS Nano; collaboration : Prof. Jun Lou, Rice University.
- Plasmo-electronics
Effective intermediaries for the transformation of electromagnetic energy into electrical energy are surface plasmons. Actually, a network of self-organized nanoparticles or a semiconductor quantum well's electrical conduction is facilitated by the hot carriers photogenerated by plasmonic excitations. We are investigating these phenomena experimentally (spectroscopy and local imaging of optical responses and charge transport) as well as theoretically (plasmon/charge carrier conversion, charge transfer by hybridization of molecular/metallic states, tunneling or photo-thermo-induced transport). New applications are made possible especially in the area of sensors (resistive and capacitive).
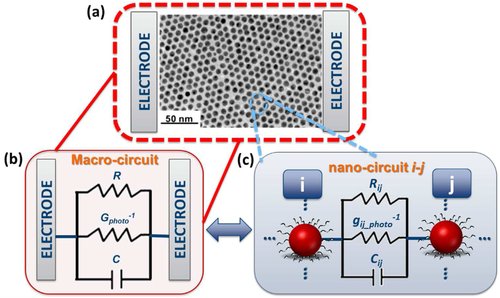
Plasmo-electronics in hybrid self-assembled molecule/nanoparticle structures. The photocurrent generated by resonant excitation of nanoparticle surface plasmons is studied as a function of physical parameters (nanoparticle size, shape, nature of the stabilising molecule) and applied stresses (AC electric field, substrate deformation, temperature). Published in Materials Today Nano; collaboration : Prof. Jérémie Grisolia LPCNO-INSA, Toulouse.
Coded adaptative hyperspectral imaging
Hyperspectral imaging, or simply spectral imaging, measures the light, infrared and sometimes UV spectrum for each point in a 2D scene. It thus provides a hyperspectral cube rich in information, which is used for a wide range of applications (environmental monitoring, urban planning, precision agriculture, anomaly detection, etc.). Exhaustive measurement of this cube is time-consuming, and generates a lot of data that needs to be stored, transmitted and post-processed. For a very large number of applications, it is possible to obtain the information of interest (CO2 levels, spectral classification, water stress, etc.) without using the entire cube.
The aim of our work is to exploit a few controlled spectral mix acquisitions, so-called "coded" acquisitions, to reduce the amount of information at acquisition and throughout the processing chain to extract the information of interest.
Our approach is based on the co-design of the device used to measure coded acquisitions, a DD-CASSI (Double-Disperser Coded Aperture Snapshot Spectral Imager) with programmable coded mask [hal-01182610, hal-02523018, tel-03997931] and the associated processing algorithms [tel-03404224, hal-02993037, hal-03610209].
We have thus been able to demonstrate experimentally the reduction by more than an order of magnitude in the number of measurements required to extract the entire hyperspectral cube [hal-03610209], as well as neural network-based approaches for spectral classification directly from 2 coded acquisitions instead of using the entire hyperspectral cube (containing more than 100 spectral bands) [hal-03339620].
Back to Photonic page.